The Evolution of Car Battery Technology From Lead-Acid to Lithium-Ion
The Evolution of Car Battery Technology From Lead-Acid to Lithium-Ion - Lead-Acid Batteries The Pioneers of Automotive Power
Lead-acid batteries, a pioneering invention of Gaston Planté in 1859, were the first rechargeable batteries ever developed. This breakthrough laid the groundwork for the integration of electricity into automotive power, marking their status as the original driving force behind early vehicles. While the rise of lithium-ion batteries has shifted the focus in recent times, lead-acid technology continues to be indispensable in many applications. Their role in providing power for starting, lighting, and ignition systems in conventional vehicles remains essential. Moreover, the existence of variations like gel, AGM, and VRLA batteries demonstrates their adaptability to various needs. Ongoing research strives to overcome inherent performance limitations of lead-acid batteries, such as improving their energy capacity and lifespan, while simultaneously ensuring they continue to serve reliably as backup power sources and integral parts of hybrid vehicle systems. Lead-acid batteries stand as a testament to the early days of electric mobility and serve as a reminder of their critical impact on the progression of modern automotive power. Their enduring relevance is undeniable, even in a landscape dominated by newer technologies.
Lead-acid batteries, a product of Gaston Planté's ingenuity in 1859, were a revolutionary breakthrough—the first batteries capable of storing and releasing energy repeatedly. This foundational discovery laid the groundwork for automotive power systems as we know them, dominating the field for well over a century. Their operation relies on the interplay between lead dioxide and sponge lead immersed in sulfuric acid, generating a nominal voltage of about 2 volts per cell.
While these batteries are notably heavier than some modern alternatives, they excel at delivering high bursts of energy, making them well-suited for starting combustion engines. Their capacity to handle rapid discharge is essential for the initial power demands of engine ignition. However, their lifespan is relatively limited compared to newer technologies, typically enduring between 300 to 700 charge-discharge cycles depending on how deeply they are discharged and the surrounding environment.
Their efficiency, while adequate for many applications, is not without its shortcomings. Lead-acid batteries convert energy with a 70% to 85% efficiency, losing a significant portion as heat. This inefficiency is a factor that needs careful consideration in any system focused on energy optimization.
Over time, lead-acid technology has seen advancements, such as the AGM design. These variations improve performance, reduce maintenance needs, and generally bolster safety compared to traditional designs that rely on a liquid electrolyte. This shows how even older technologies can be refined and improved for greater utility. Economically, lead-acid batteries remain a competitive choice, especially in more affordable vehicle classes, as their production cost is lower compared to Lithium-ion batteries.
However, they suffer from a notable vulnerability to temperature fluctuations. Performance declines in both very cold and very hot conditions, highlighting a reliability concern in diverse environments. It’s remarkable how a technology initially intended for stationary applications has been engineered into a crucial component of modern automobiles, demonstrating significant adaptive design.
Despite the rise of lithium-ion technology, lead-acid batteries continue to find their niche in the automotive world. They are especially crucial in systems like start-stop engines where they provide essential power, proving that even older technologies can be successfully integrated into novel applications. This underscores that while the evolution of battery technology is ongoing, the legacy of lead-acid batteries remains significant. They serve as a reminder of how foundational inventions can adapt and remain relevant even as the field undergoes constant change.
The Evolution of Car Battery Technology From Lead-Acid to Lithium-Ion - Nickel-Metal Hydride Batteries Doubling the Range
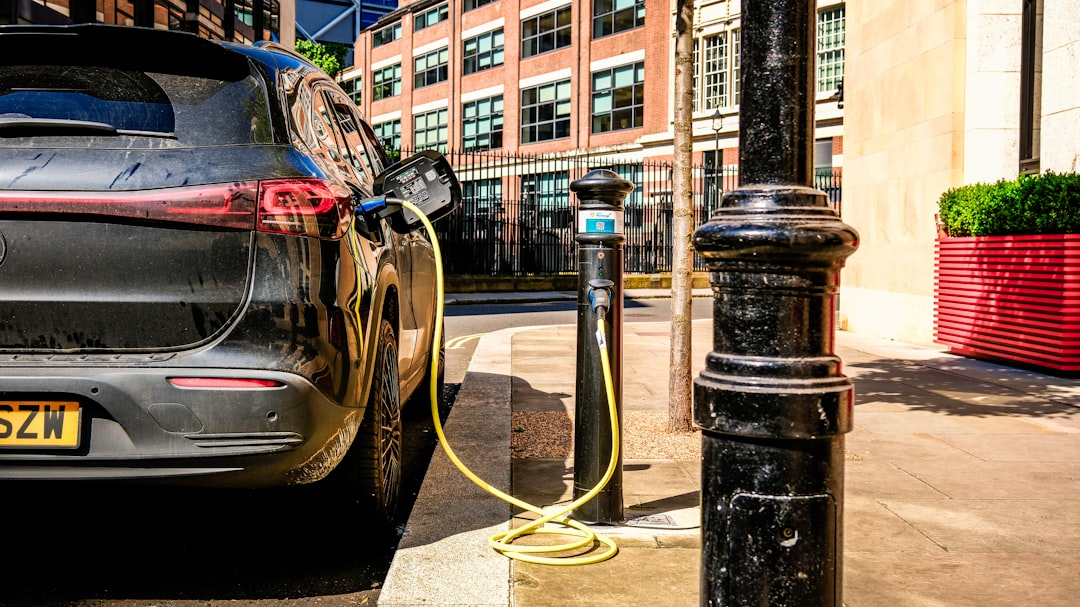
Following the lead-acid era, nickel-metal hydride (NiMH) batteries emerged as a significant step forward in automotive power. These batteries offered a more compact and lighter alternative, with the ability to store more electrical energy than their lead-acid predecessors. This improvement paved the way for the development of early hybrid and electric vehicles, as they enabled increased driving range compared to vehicles reliant solely on lead-acid power.
However, the market adoption of NiMH technology was initially slow. Despite offering a clear performance gain over older battery technologies, they struggled to gain widespread acceptance. The reasons for this are likely multifold and related to cost, availability, and possibly some remaining technical hurdles that needed to be overcome.
Subsequently, lithium-ion batteries have largely supplanted NiMH batteries in the majority of electric vehicles due to their superior energy density and quicker charging times. Lithium-ion's advantages in these areas resulted in better range and a more convenient user experience.
Nevertheless, NiMH batteries continue to find niches where their characteristics are a better fit, such as in some specific hybrid designs or certain power tools. This illustrates that despite the rapid advancements in battery technology, the development of other chemical solutions remains important for optimizing the energy efficiency and lifespan of power systems across a variety of applications. While NiMH technology may not have become the dominant choice, it contributed significantly to the ongoing progress towards more efficient electric powertrains. Their role in the evolution of automotive batteries underscores that the quest for optimal battery chemistry for specific applications is an ongoing endeavor, highlighting both the advancements and the continuing need for improved performance across all areas of electric vehicle development.
Nickel-metal hydride (NiMH) batteries represented a substantial leap forward in electric vehicle battery technology, surpassing the limitations of lead-acid batteries in terms of size, weight, and energy storage capacity. While they initially sparked the development of hybrid and electric vehicle markets, their broader adoption was initially hampered by various factors.
NiMH batteries, while capable of storing more energy in a smaller package, have a lower energy density compared to lithium-ion batteries, typically around 100-140 Wh/kg versus 150-250 Wh/kg. However, it's worth noting that advancements in NiMH chemistry and design are pushing these boundaries. One notable advantage of NiMH is their wider operational temperature range, functioning efficiently from -20°C to 60°C. This makes them a potentially attractive choice for vehicles operating in diverse climates. Recent breakthroughs in NiMH technology suggest a doubling of range compared to older designs, potentially impacting future electric vehicle development significantly.
Furthermore, NiMH generally exhibit a greater level of safety due to a lower risk of thermal runaway compared to lithium-ion counterparts. This makes them inherently safer for automotive applications where fire or explosion hazards need to be minimized. A notable feature of modern NiMH batteries is their reduced self-discharge rate, a considerable improvement over earlier versions. This lower self-discharge rate means less energy is lost over time when the battery is not in use.
Moreover, NiMH batteries boast a longer cycle life in comparison to lithium-ion batteries, especially in applications with less intense charging cycles, like those found in many hybrid vehicle configurations. The production costs for NiMH batteries also remain relatively competitive compared to lithium-ion batteries, owing to the abundance of nickel and a generally simpler manufacturing process. This cost advantage can be crucial for manufacturers seeking to balance performance and affordability.
However, NiMH batteries aren't without limitations. A phenomenon known as the "memory effect" can lead to reduced usable capacity if only partially discharged and recharged consistently. Understanding and managing this memory effect is vital to ensure optimal performance. Additionally, though less common, hybrid systems are being researched that would incorporate both NiMH and lithium-ion batteries. The goal is to combine the strengths of both technologies to create vehicles with extended range, enhanced safety, and optimized manufacturing costs. While lithium-ion has become dominant in most modern electric vehicles, the development and research around NiMH continue to hold promise for specialized or future applications, as they provide a comparatively safer and more robust alternative for certain use cases.
The Evolution of Car Battery Technology From Lead-Acid to Lithium-Ion - Lithium-Ion Batteries Revolutionizing Electric Vehicles
Lithium-ion batteries have revolutionized electric vehicle (EV) technology, offering a significant upgrade compared to earlier battery types like lead-acid and nickel-metal hydride. Their enhanced energy density and efficiency have made them the dominant power source in EVs, leading to a substantial surge in demand, especially noticeable in the Chinese EV market. The development of lithium-ion batteries has involved innovations like incorporating cobalt into the cathode to boost stability and energy storage capacity. However, this has also raised concerns about cobalt mining's environmental impact, stimulating research into cobalt-free battery alternatives.
Despite improvements, the cost of lithium-ion batteries remains a hurdle, contributing significantly to the overall expense of electric vehicles (around 50%). However, continuous research and development efforts focus on enhancing battery performance and driving down costs, which are key factors in expanding EV adoption. Looking ahead, the future of lithium-ion batteries in the EV landscape is promising. Research and development promise better energy storage capabilities and more efficient EV powertrains, hinting at further breakthroughs that could reshape the automotive industry in the years to come.
Lithium-ion batteries have emerged as the dominant power source for most electric vehicles (EVs), representing a substantial leap forward compared to earlier technologies like lead-acid batteries. This shift is driven by their superior performance characteristics, particularly their increased energy density and efficiency. The demand for these batteries in the EV sector has skyrocketed, with a 65% increase in 2022 alone, largely fueled by the growing popularity of electric passenger cars. This surge is particularly evident in regions like China, where demand climbed by over 70% during the same period.
The foundation of lithium-ion battery operation lies in the movement of lithium ions between a positively charged cathode and a negatively charged anode, a process that enables the battery to be recharged. Many modern lithium-ion batteries incorporate cobalt into the cathode to enhance stability and energy density. However, the reliance on cobalt has sparked growing research into developing alternative battery chemistries that don't rely on this element.
Unfortunately, this advancement comes with a cost. The production of lithium-ion batteries accounts for about 50% of the total cost of an EV, making them currently more expensive than conventional vehicles using fossil fuels. This cost barrier can influence consumer decisions and highlights the ongoing need for improvements in manufacturing efficiency and material acquisition.
Despite the cost factor, the increasing adoption of EVs has been driven by both lithium-ion battery advancements and other factors, such as government incentives aimed at promoting cleaner transportation and growing environmental concerns about traditional vehicle emissions. These trends suggest that the future of transportation might involve a greater reliance on electric powertrains.
The field of lithium-ion battery research is far from stagnant. Scientists and engineers are continuously exploring ways to improve the technology. This includes developing novel electrode materials, tailoring batteries for diverse applications (e.g., specific vehicle classes or climate conditions), and evaluating alternatives like lithium-metal batteries for EVs.
Looking toward the future, the vision for lithium-ion battery technology in the coming years centers around improvements in energy storage and performance. The ability to achieve longer range and faster charging times on a single charge could dramatically impact the EV market, making electric vehicles even more appealing and possibly leading to widespread adoption. While lithium-ion batteries are currently dominating the scene, it's important to recognize the ongoing investigation into future technologies like lithium-sulfur and lithium-air batteries. These potential successors promise substantial advancements in energy density, but face challenges in becoming commercially viable. It's exciting to contemplate the future of electric vehicles and the battery innovations that will continue to shape their capabilities and influence.
The Evolution of Car Battery Technology From Lead-Acid to Lithium-Ion - Energy Density Improvements Key to Longer Driving Distances
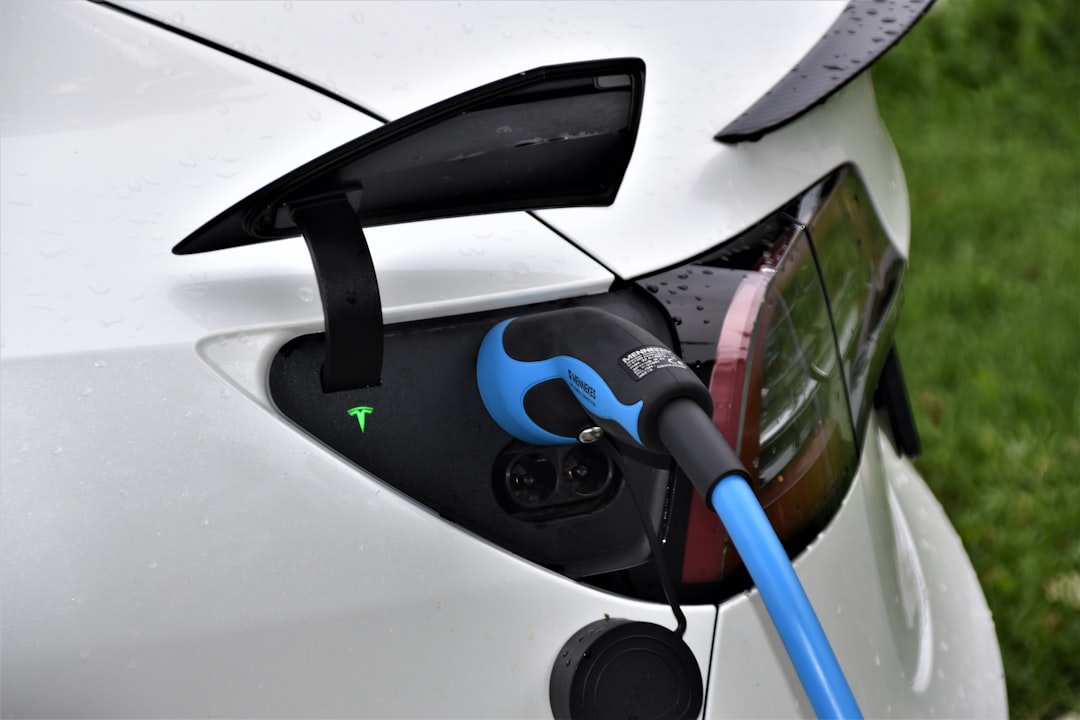
Improving the energy density of electric vehicle batteries is a crucial step toward longer driving ranges and a more convenient user experience. The increasing popularity of electric vehicles is pushing the field of lithium-ion battery development forward, with researchers working to significantly increase energy density. Estimates suggest that top-performing batteries could achieve an energy density between 600 and 800 Wh/kg by 2030. This level of performance has the potential to drastically increase the distance an electric vehicle can travel on a single charge and potentially reduce the time required to recharge. However, there are persistent hurdles related to reducing the size and weight of batteries while maintaining their safety and energy density. The pursuit of next-generation battery technologies, including solid-state and lithium-air batteries, are essential steps in addressing these challenges. The potential for these technologies to meet or exceed expectations will significantly impact the future of electric mobility.
The journey towards longer driving distances in electric vehicles (EVs) is intrinsically linked to advancements in energy density. Lithium-ion batteries, the current workhorse of the EV sector, have demonstrably outperformed earlier technologies like nickel-metal hydride (NiMH). NiMH batteries typically achieve energy densities around 100-140 Wh/kg, while lithium-ion easily surpasses 250 Wh/kg, leading to a roughly two- to threefold improvement in energy efficiency. This direct correlation between energy density and driving range is a significant factor pushing lithium-ion to the forefront of EV power.
Furthermore, rapid charging capabilities are rapidly evolving within lithium-ion battery technology. Recent innovations now enable charging to 80% capacity in under 30 minutes, a huge leap forward that addresses one of the major barriers to EV adoption, commonly referred to as 'range anxiety'. Reducing charging times provides a much more user-friendly experience, thus incentivizing broader use of electric cars.
However, the road to optimal EV performance is not without its obstacles. One challenge facing lithium-ion technology is maintaining performance across diverse temperature ranges. While these batteries offer excellent energy storage, performance can suffer in cold weather conditions, with a decrease in energy density as high as 20%. This factor underscores the continuous need for better battery management systems that ensure reliable operation in various climates.
Fortunately, lithium-ion batteries have shown significant improvements in other areas, such as self-discharge. While older technologies like lead-acid suffer from a relatively high self-discharge rate of around 20%, modern lithium-ion batteries boast impressively low self-discharge rates in the range of 2-5% per month. This means that the majority of the stored energy is readily available when the vehicle needs it, even after a period of non-use.
There's also been notable progress in extending the lifespan of lithium-ion batteries. When properly managed, these batteries can withstand more than 1500 charge-discharge cycles, a vast improvement over the 300 to 700 cycles that lead-acid batteries typically deliver. This increased durability directly contributes to the overall longevity of the EVs they power.
The quest for enhanced energy density and safety continues to drive research into alternative cathode materials. Lithium iron phosphate (LiFePO4) and manganese-rich compounds are being explored for their potential to offer safer operating conditions and better thermal stability. These materials could eventually lead to higher energy densities while reducing reliance on cobalt, a mineral with associated environmental concerns.
Furthermore, as lithium-ion battery technology matures, initiatives centered around recycling are growing. The industry acknowledges the need to efficiently recover valuable elements like lithium, cobalt, and nickel from discarded batteries. This is crucial not only for environmental reasons but also for lowering the overall life-cycle cost of lithium-ion batteries.
Efforts are also underway to integrate lithium-ion and solid-state battery technologies into hybrid systems. The pursuit of these hybrid approaches stems from the hope that they can address safety concerns related to lithium-ion while offering even higher energy densities and potentially faster charging.
While the technological benefits are numerous, it's important to recognize that the cost of lithium-ion batteries, which typically accounts for roughly half the total cost of an EV, poses a significant hurdle to broader EV adoption. The future of widespread electric vehicle utilization will likely rely on achieving both production efficiency and sourcing innovation, thus driving down battery costs to a more competitive level.
However, the ever-increasing global demand for EVs, fueled by environmental considerations and government incentives, has also created an unexpected challenge: concerns around the sustainability of the lithium supply. As the number of electric cars increases, the industry faces the challenge of ensuring a reliable and secure source of lithium. Research into alternative materials and extraction methods is becoming increasingly important to guarantee a consistent supply of battery components for future production needs.
The advancement of energy density in lithium-ion batteries holds immense potential for the future of transportation. While challenges persist, ongoing research and innovation in diverse areas continue to pave the way for a cleaner, more sustainable automotive landscape. The interplay between innovation and market forces is a constant process. It is exciting to witness how these advancements continue to shape the evolution of EV technology.
The Evolution of Car Battery Technology From Lead-Acid to Lithium-Ion - Faster Charging Technologies Reducing Downtime
Faster charging is transforming how we interact with electric vehicles (EVs) by drastically reducing the time it takes to replenish their batteries. This translates to less downtime for EV users, making them a more viable everyday option. Lithium-ion battery advancements have made charging to 80% capacity in as little as 10 minutes a reality in some labs, with ambitious targets for even quicker rates. However, a lot of current EV models haven't reached this level of speed, with a typical time to 80% being closer to 18 minutes, suggesting there's still a lot of room for improvement in battery technology and charging infrastructure. New approaches like inductive charging and smarter ways to manage charging infrastructure are stepping up to meet the demands of the growing electric mobility market. Addressing the time it takes to charge EVs, also called 'downtime', is crucial for widespread EV adoption, making them more user-friendly and convenient for daily use. As research progresses, the goal of faster and more efficient charging remains central to realizing the full potential of electric vehicles in our transportation future.
The quest for reducing downtime during EV charging is a significant driver in the ongoing development of faster charging technologies. While a 15-minute charge to 80% capacity is the ideal as defined by the US Advanced Battery Consortium, even cutting-edge EVs like the Porsche Taycan still fall short of that target, needing roughly 18 minutes. Nonetheless, impressive progress is being made. Certain systems now achieve 350 kW charging rates, enabling EVs to reach 80% in less than 20 minutes. This is a huge leap forward from the charging times of just a few years ago, though this is also a complex area affected by several factors that may prevent widespread adoption.
The pursuit of faster charging involves significant changes in battery materials and configurations. Researchers are experimenting with materials like LiFePO4 and solid-state electrolytes to enhance ion mobility, which could potentially double the charging speed. Integrating supercapacitors within battery packs presents a hybrid approach that leverages their quick energy absorption and release. This could dramatically improve aspects like regenerative braking and acceleration, but we still have much to learn about how to best integrate these two technologies, and this will be important to the future of EV adoption.
Efficiently managing battery temperature during the charging process is another crucial aspect of this work. More sophisticated thermal management, like enhanced liquid cooling, enables faster charging rates while minimizing risks to the battery's health and longevity. The development of “smart” charging stations that incorporate Internet of Things (IoT) principles is gaining traction. These stations could optimize charging schedules, not only based on vehicle needs but also on grid stability and energy demands. However, the complexity of integrating large-scale IoT systems on a reliable level is one of the challenges that needs to be solved before this vision is realized.
Furthermore, bidirectional charging is becoming more prevalent. Equipped with this feature, EVs can not only draw power from the grid but also send power back, leading to greater efficiency and cost reductions. Battery swap technologies remain a more niche approach, but they're of great interest for fleet operators and urban environments where quick refueling is essential. But a critical part of this approach is expanding the network of swap stations to the point where it is widely available and can effectively reduce or eliminate range anxiety in drivers.
Modern Battery Management Systems (BMS) play a vital role in extending battery lifespan and optimizing charging cycles using machine learning approaches. These systems can prolong the useful life of batteries, making it less likely that users will encounter downtime due to battery degradation. The infrastructure needed to support fast charging is also being developed. The growth of high-power Direct Current (DC) fast charging stations has a large impact on EV charging, allowing for quicker charging times compared to older Alternating Current (AC) options.
The potential of wireless charging, through integrated pads, is an interesting development for the future. This could make charging as simple as parking in a designated spot, potentially removing any downtime associated with actively connecting a charging cable. However, the challenges associated with efficiency, cost, and power delivery in this context might take some time to be properly addressed before it is widely adopted. The evolution of battery technology is crucial to reducing range anxiety and driving greater EV adoption. Faster charging promises a future where EVs can compete more effectively with traditional fuel-powered vehicles. Though significant challenges and hurdles remain, progress in this sector is being made, and as it does, EV ownership might become a more realistic choice for a growing number of people.
More Posts from :