The Science Behind Class A Fire Extinguishers Breaking Down How They Combat Wood, Paper, and Fabric Fires
The Science Behind Class A Fire Extinguishers Breaking Down How They Combat Wood, Paper, and Fabric Fires - Water Based Solutions The Core Technology Behind Class A Extinguishers
The fundamental principle behind Class A fire extinguishers lies in their water-based extinguishing agents. These extinguishers are specifically designed to tackle fires involving everyday combustible materials like wood, paper, and textiles. The core mechanism involves using water's inherent ability to absorb heat and cool down burning substances. This cooling action, coupled with the water's capacity to saturate the burning material, effectively smothers the flames and prevents rekindling.
However, this simplicity doesn't mean that Class A extinguishers are universally applicable. It's crucial to remember that using water on certain types of fires, notably those involving oil or grease, can be counterproductive and potentially worsen the situation.
The technology behind water-based firefighting has undergone refinements and innovations over time, particularly in places like Australia and Europe. These advancements aim to optimize the effectiveness of water-based suppression systems, making them even more reliable tools for combating fires involving ordinary combustible materials. While these improvements offer promise, the basic principles of water-based extinguishing remain central to Class A fire suppression.
The core technology behind Class A extinguishers relies on the fundamental properties of water. Its ability to absorb heat is central to its effectiveness. When water hits a burning material like wood or fabric, it rapidly absorbs the heat, reducing the temperature below the point where combustion can sustain itself. This cooling effect is crucial, especially considering the intense temperatures these fires can reach, often exceeding 1,500 degrees Fahrenheit.
While primarily a cooling agent, water also contributes to fire suppression by producing steam. This steam displaces oxygen, suffocating the flames, which is especially helpful in controlling rapidly expanding fires. These extinguishers are engineered for a specific discharge range, typically 30 to 40 feet, allowing for targeted application from a safe distance.
However, the inherent characteristics of water can also present challenges. For instance, its natural surface tension can impede penetration into porous materials. To counter this, various additives are often incorporated into the water solution to lower surface tension, thus promoting better penetration into materials like wood and fabric.
Interestingly, water's effectiveness is also related to its exceptionally high heat capacity. This translates to its ability to absorb a considerable amount of heat before its own temperature increases, extending its firefighting duration. Furthermore, in contrast to chemical-based extinguishers, water leaves no residue, making it ideal in environments where cleanliness is paramount.
It is important to note that water extinguishers are not universally suitable. Water's freezing point restricts their application in extremely low temperatures, though some formulations use antifreeze additives to mitigate this. Additionally, while water is generally non-toxic, improper use, such as on electrical or oil-based fires, can be dangerous. Precisely understanding the nature of the fire is critical for ensuring the proper application of a water-based extinguisher.
Finally, the internal pressure of these extinguishers is typically around 100-125 psi, which is instrumental in delivering a powerful and effective water stream. This pressure also contributes to the creation of a fine mist, enhancing the cooling and coverage across the affected area. This complex interplay of physical properties makes water an effective, yet nuanced, tool in controlling Class A fires.
The Science Behind Class A Fire Extinguishers Breaking Down How They Combat Wood, Paper, and Fabric Fires - Breaking Down The Science of Heat Absorption in Wood Fires
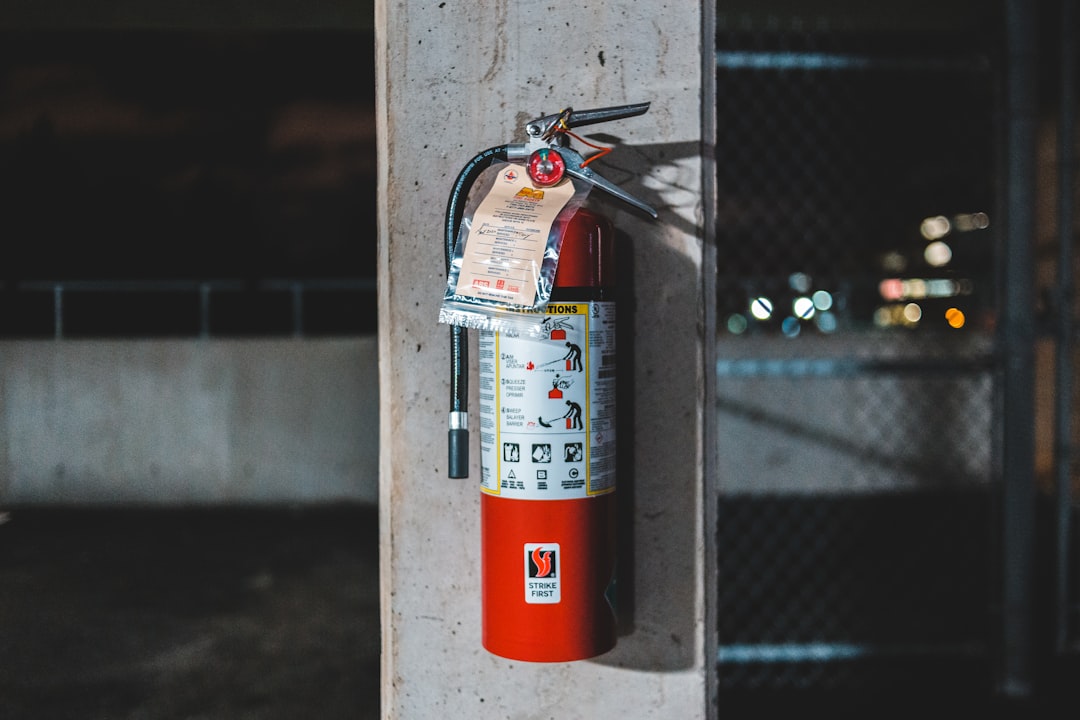
Delving into the science of heat absorption in wood fires reveals a complex interplay of chemical and physical processes. Wood's combustion unfolds in distinct stages: ignition, pyrolysis, and ultimately, combustion. Each phase contributes to the overall fire behavior and the production of gases and smoke, which is critical for comprehending the fire's progression.
Pyrolysis plays a crucial role, occurring when wood encounters high temperatures without sufficient oxygen. During pyrolysis, the wood breaks down, releasing flammable gases and contributing to the fire's intensity and spread. This process, alongside the expansion of heated air within the burning wood, generates pressure, leading to the characteristic crackling sounds associated with wood fires.
Importantly, different wood types exhibit varied burning patterns, influencing their responses to external heat sources. These differences, combined with the inherent chemical composition of wood, impact the efficiency of firefighting methods. A thorough understanding of these factors is essential for developing effective fire management strategies and applying appropriate fire suppression techniques, especially for Class A fire extinguishers, which are specifically designed to combat fires involving materials like wood, paper, and fabric. Without a clear grasp of these wood fire dynamics, achieving successful fire suppression can be challenging.
Wood, being a complex material composed of various interacting constituents, behaves in a specific way when exposed to fire. The process of wood combustion involves several stages, including ignition, pyrolysis, combustion, and eventual extinction – a sequence well-documented in the field of fire science. Pyrolysis, a key chemical reaction, occurs when wood is exposed to high temperatures in the absence of oxygen, releasing heat, gases, and smoke. This process generates an expansion of heated air within the burning material, resulting in the familiar popping sounds associated with wood fires due to pressure differentials.
Different wood types exhibit unique burning behaviors and intrinsic self-extinguishing characteristics, especially when exposed to external radiant heat sources. Class A fire extinguishers are designed for tackling fires involving common combustibles like wood, paper, and textiles. Their primary extinguishing mechanism relies on the cooling properties of water or foam. By absorbing heat and lowering the temperature of the burning material below its ignition point, these agents effectively disrupt the combustion process.
However, the flammability of wood in construction environments presents considerable sustainability concerns due to its relatively low ignition temperature and sustained combustion characteristics. Understanding the dynamics of fire, including the influence of moisture content, and material type, is critical for effective fire suppression. Firefighters require a deep understanding of these fire behaviors to accurately assess each fire situation. It's notable that, under specific radiation conditions, wood fires can exhibit a "near-limit blue flame" phenomenon, which illustrates a distinctive burning behavior tied to external energy inputs.
Water’s high specific heat capacity, around 4.18 joules per gram per degree Celsius, plays a central role in extinguishing wood fires. This capacity enables water to absorb significant heat before its temperature increases dramatically, making it vital for cooling the intense heat generated by wood fires that can reach well over 1500°F (815°C). The conversion of water into steam when applied to a fire further aids suppression. Steam expands to over 1600 times its liquid volume, displacing oxygen and suffocating the flames.
The phenomenon of latent heat, the energy needed for water to transition from liquid to vapor without a temperature change, significantly contributes to fire suppression. It allows water to continuously absorb heat during the vaporization process. Interestingly, a higher moisture content in wood can paradoxically lead to a slower fire progression, challenging the assumption that dry wood is always more dangerous. Water's relatively high boiling point (100°C at sea level) enables sustained heat absorption until it converts to steam, further delaying the fire's ability to re-ignite when water is used.
Certain additives help lower water's surface tension, increasing penetration into the porous structures of wood, enhancing its effectiveness. Achieving a temperature below the wood's ignition point (roughly 400-600°F) is essential for disrupting the chemical reactions that sustain combustion. The internal pressure within fire extinguishers also influences water's effectiveness, as higher pressures lead to a finer mist that enhances heat absorption and coverage. While water is generally considered non-toxic and residue-free, it's crucial to understand its limitations. Misusing water on inappropriate fires, like those involving flammable liquids, can create significant hazards, including re-ignition or explosions. The multifaceted nature of water, including its ability to absorb heat, convert to steam, and penetrate material, makes it a valuable tool for fire suppression, but its limitations must be considered.
The Science Behind Class A Fire Extinguishers Breaking Down How They Combat Wood, Paper, and Fabric Fires - Chemical Reactions Between Fire Retardants and Paper Materials
Paper, a frequent contributor to Class A fires, benefits from fire retardants that alter its combustion behavior. These chemical agents work by influencing the way paper burns, often by generating a protective layer of char or by decomposing the paper's polymers into less flammable substances. Some retardants activate a series of chemical reactions when exposed to high temperatures, leading to intumescence, where the material expands and creates a heat-resistant barrier that shields the paper from the flames. While these reactions can significantly slow down ignition and the spread of fire, concerns regarding the environmental impact and the release of potentially harmful chemicals from some fire retardants remain. Understanding the specifics of these chemical reactions is vital for developing effective fire safety strategies and improving the fire-resistant properties of materials across various uses. The goal is to minimize damage and risk in instances of fire, while considering the overall environmental consequences of using these chemicals.
When it comes to protecting paper materials from fires, we often rely on chemical fire retardants. These substances work through a variety of chemical interactions that alter the way paper burns. For instance, specific types of retardants, like those based on ammonium phosphate or boron compounds, can engage with the cellulose in paper fibers, forming a layer of char. This char acts as a barrier that resists further burning, slowing the spread of fire.
The thermal breakdown of paper is also influenced by fire retardants. Some retardants can actually lower the temperature at which paper begins to release flammable gases, effectively making the material less likely to catch fire easily. Additionally, certain retardants work by absorbing heat when exposed to flames – a process known as an endothermic reaction. This heat absorption cools the paper and surroundings, potentially delaying ignition and preventing the fire from quickly spreading.
The protective char layer formed by fire retardant reactions provides a significant obstacle to combustion. It acts as a shield, reducing the surface area exposed to flames and decreasing the risk of sustained burning. This, in turn, allows more time for evacuation and response to fires.
Beyond the char formation, some fire retardants disrupt the combustion process in a different way. They interfere with free radicals, reactive molecules that play a critical role in maintaining the chain reactions of fire. By targeting these radicals, certain retardants can effectively break the chain reaction in paper, suppressing the fire.
Another fascinating mechanism involves intumescent fire retardants. When heated, these types of retardants expand and foam, creating a protective barrier that inhibits the release of flammable gases. This reduces the paper's overall flammability, preventing the fire from spreading or escalating quickly.
It's important to note that the effectiveness of a retardant can be influenced by the moisture content of the paper. In moist conditions, the normal chemical reactions between retardant and cellulose can be altered, potentially leading to decreased efficacy.
Moreover, the benefits of fire retardants can be further enhanced when they are combined with other materials, such as different fibers or fillers. This blending often creates a synergistic effect, resulting in compounds that offer superior fire resistance compared to using the retardant alone. For instance, mixing cellulose with a mineral-based retardant can increase thermal stability, potentially improving fire resistance.
However, there are downsides to consider. When paper treated with fire retardants is exposed to fire, a residue may be left behind that can hinder its recyclability. This raises concerns about the long-term environmental impact of retardant use and the need to carefully evaluate the potential downsides.
Finally, the selection of fire retardants often hinges on the specific requirements outlined in fire safety regulations. Compliance with standards related to the efficacy and safety of retardants can impact the choice of which retardant is used in the paper products we encounter in building construction, décor, and packaging materials. Understanding these regulations is essential in the quest to create fire-safe materials that meet both practical needs and safety concerns.
The field of fire retardant chemistry is constantly evolving, with researchers striving to develop new materials and approaches that are both effective and environmentally sustainable. As our understanding of these complex interactions deepens, we can continue to develop innovative and safer solutions for managing fire risks in various applications.
The Science Behind Class A Fire Extinguishers Breaking Down How They Combat Wood, Paper, and Fabric Fires - Understanding Oxygen Deprivation Methods for Fabric Fires
Fabric fires, often part of Class A fire scenarios, are effectively tackled by methods that limit oxygen availability. This stems from the fundamental principle that fire requires oxygen to sustain combustion. By reducing or eliminating oxygen access, the chemical reactions that drive the fire are disrupted, extinguishing the flames.
Common tactics involve deploying agents such as foam or carbon dioxide. Foam works by forming a blanket over the fire, isolating it from the surrounding air, which is the primary source of oxygen. Carbon dioxide, on the other hand, displaces the oxygen in the immediate area, effectively starving the fire. The effectiveness of these methods is particularly notable in fabric fires, as these materials can ignite quickly and maintain a vigorous burn.
Understanding these oxygen deprivation techniques is essential for fire safety. Employing them effectively reduces the hazards associated with fabric fires, potentially mitigating damage and loss of life. As such, a deep understanding of fire chemistry and the practical application of oxygen deprivation methods are crucial elements of comprehensive fire safety education.
When tackling fabric fires with Class A extinguishers, understanding how they interrupt the combustion process is vital. One key mechanism is **oxygen displacement through steam generation**. As water hits the fire, it rapidly vaporizes, creating a significant volume expansion (over 1600 times its liquid state). This steam effectively pushes oxygen away from the flames, suffocating the fire.
Fabric fires, like those involving cotton or wool, typically need temperatures around 410°F to 600°F to ignite. The effectiveness of water lies in its ability to quickly reduce the temperature below this threshold, preventing rekindling. This is further enhanced by the phenomenon of **thermal layering** where hot gases rise, creating distinct layers. Applying water strategically can disrupt these layers and the convection currents that sustain the fire.
However, water's **surface tension** can impede its penetration into porous fabrics. To overcome this, surfactants are often added to water-based extinguishers to lower surface tension, allowing for better water distribution and absorption within the fabric's fibers.
Furthermore, many fabrics are treated with **flame retardants**. These chemicals work by changing the chemical reactions involved in combustion, sometimes by creating a char layer that inhibits heat transfer. The presence of these retardants extends the time it takes for a fire to spread.
It's also crucial to consider that **fabric composition** plays a significant role in how it burns. Natural fabrics, like cotton, often ignite more readily than synthetic ones like polyester, which may melt or drip instead. Understanding these material differences is critical for choosing the appropriate extinguishing technique.
Despite seemingly extinguishing a fabric fire, the possibility of **rekindling** always exists if residual heat remains. Thorough cooling and water penetration are necessary to ensure complete extinguishment, especially with materials that tend to smolder like upholstery.
The **moisture content** of the fabric can also influence its fire behavior. Interestingly, while we might assume dry fabrics are more flammable, wetter fabrics may actually resist ignition initially. Understanding this complex relationship is key to using water-based extinguishers effectively.
Although primarily a cooling agent, water can also engage in **exothermic reactions** with some combustible materials, further absorbing heat and hindering fire spread.
The **internal pressure** within Class A extinguishers, typically around 100-125 psi, is also a crucial factor. This high pressure allows the water to be discharged as a fine mist, significantly improving the distribution and cooling capacity across a wider area, maximizing effectiveness in extinguishing fabric fires.
All these factors contribute to a nuanced understanding of how Class A extinguishers tackle fabric fires. While the basic principle of cooling is fundamental, there's a fascinating interplay of physical and chemical processes that make effective fire suppression possible.
The Science Behind Class A Fire Extinguishers Breaking Down How They Combat Wood, Paper, and Fabric Fires - Pressure Systems and Spray Patterns in Class A Extinguishers
Class A fire extinguishers rely on pressurized systems to deliver their extinguishing agents effectively. The internal pressure, typically maintained at 100-125 psi, is key to generating a powerful and wide-reaching spray, often covering a distance of 30 to 40 feet. This pressure is crucial for achieving an optimal spray pattern, often a fine mist, which maximizes the cooling effect and spread of the extinguishing agent across the burning area. The finer mist produced under pressure enhances heat absorption and facilitates more efficient fire suppression. Furthermore, the engineered spray patterns themselves are designed for optimal coverage and effectiveness. This optimized spray pattern allows for targeted application and minimized risk of rekindling. Understanding these pressure and spray dynamics is crucial for the successful use of Class A extinguishers, especially in settings where combustible materials like wood, paper, and fabrics are common.
Class A extinguishers, while seemingly simple in their application of water, rely on intricate pressure systems and spray patterns to effectively combat fires involving ordinary combustibles like wood, paper, and fabrics. The internal pressure, typically ranging from 100 to 125 psi, serves a dual purpose. Not only does it propel the water, but it also plays a critical role in establishing the optimal spray pattern, balancing reach with a wide dispersion for efficient coverage.
The design of the nozzle significantly impacts the spray pattern and, most importantly, the size of the water droplets. A well-designed nozzle can create a fine mist, which maximizes the surface area of the water in contact with the heat source. This contrasts with a solid stream of water, where a much smaller surface area is exposed to the heat, thereby significantly impacting the rate and efficacy of cooling. The smaller droplets in a fine mist evaporate more readily, maximizing heat absorption and accelerating the cooling process, which is crucial for rapidly escalating fires.
Interestingly, directing the spray at a particular angle can further enhance the effectiveness of water application, especially when dealing with fires involving materials like fabric or paper. A strategic angle allows the water to permeate the material, potentially preventing further fire spread.
The conversion of water to steam, facilitated by the internal pressure of the extinguisher, absorbs significant amounts of heat—this is the crucial concept of latent heat. The ability of high-pressure systems to generate steam more efficiently further enhances the displacement of oxygen, thereby suffocating the flames.
However, water's natural surface tension can sometimes impede its penetration into porous materials like wood or fabric. Fortunately, various additives can be incorporated to decrease this surface tension, allowing water to be absorbed more effectively, thus boosting extinguishing performance.
The flow rate of the water, controlled by the internal pressure, directly impacts the duration of the extinguishing effort. A higher flow rate offers the possibility of more rapid extinguishment, but it needs to be carefully managed to prevent unnecessary water damage. It's a fine balance that underscores the importance of strategic application.
The heat transfer dynamics of water shift as it's discharged, varying based on the spray pattern's specific characteristics. Thermal conductivity is altered by the pattern's design, which significantly impacts the rate at which the fire's vicinity cools.
Furthermore, environmental temperature can also impact the extinguisher's operational effectiveness. The ambient temperature can modify the water's viscosity and its heat absorption capabilities, consequently influencing the spray pattern and pressure efficacy.
In enclosed environments, backpressure from the confined space can further modify the spray pattern, resulting in a more concentrated stream. This highlights the importance of understanding the specific fire dynamics within diverse environments to ensure that extinguishers are applied optimally.
Understanding the complex interplay of factors that influence Class A extinguishers, particularly their internal pressure and spray patterns, reveals a more nuanced approach to fire suppression. It's clear that fire suppression is not a one-size-fits-all solution, and adaptability based on fire characteristics and environmental conditions is crucial for optimal efficacy and safety.
The Science Behind Class A Fire Extinguishers Breaking Down How They Combat Wood, Paper, and Fabric Fires - Temperature Control Through Advanced Cooling Mechanisms
This section explores the emerging field of advanced cooling mechanisms, with a particular focus on their potential in temperature control, especially within the context of firefighting and personal protective gear. The development of cooling textiles, employing techniques like photon-engineered radiative cooling and evaporative cooling, signifies a substantial step forward in our ability to regulate body temperature. These methods offer the promise of enhanced personal thermoregulation, potentially mitigating heat stress even in challenging environmental conditions.
While these advancements hold great promise for applications like protective gear in firefighting, the specific performance of such cooling mechanisms depends heavily on the materials used in their construction. Different fibers and polymers show variable effectiveness in dissipating heat, suggesting that further research is needed to pinpoint the most efficient materials for optimal thermal management in a range of settings. The effectiveness of these advanced cooling methods could significantly impact the safety and effectiveness of firefighting procedures, especially in the context of Class A fires.
It's worth noting that this intersection of technology and safety is particularly crucial in environments with inherent fire hazards, where the ability to regulate temperature can be critical for both personal safety and operational effectiveness. Ongoing development and testing of these cooling mechanisms is crucial to better understand their potential benefits in a variety of applications.
### Exploring Temperature Control in Fire Suppression
1. **The Power of Phase Change**: The transformation of water into steam during firefighting isn't just about cooling; it's about harnessing a massive energy shift. The energy absorbed during this phase change (around 2,260 joules per gram) significantly boosts its cooling capability compared to simply lowering the temperature of the water itself. This suggests a powerful mechanism inherent to water's nature.
2. **Micro-Droplets and Enhanced Cooling**: The fine mist created by high-pressure systems in Class A extinguishers isn't just about wider coverage. It generates a large number of small water droplets, each with a high surface area-to-volume ratio. This means more efficient heat absorption as each droplet rapidly vaporizes, potentially speeding up the cooling process. However, it remains an area for more research to truly grasp the limits of this effect.
3. **Heat Layers and Strategic Application**: The concept of thermal layering, where hot gases rise and cooler air sinks, highlights that applying water at specific angles can disrupt these layers. This creates an opportunity to more effectively cool areas at various heights within a room or enclosed space. It might be worth investigating if specific nozzle designs could further optimize this phenomenon.
4. **Surfactants: Overcoming Water's Limitations**: While water's natural surface tension can impede its penetration into porous materials, like fabrics, additives called surfactants can significantly reduce this tension. This allows water to spread and absorb more efficiently within these materials, improving the extinguisher's effectiveness. However, the long-term effects of using such additives need to be investigated more thoroughly.
5. **Moisture Content's Influence**: It's interesting that lower moisture content in fabrics can sometimes lead to faster ignition, which challenges the initial intuition that dry materials are always more dangerous. This highlights the need for a deeper understanding of how material moisture affects the ignition and combustion process.
6. **Pressure's Role Beyond Reach**: The internal pressure in a Class A extinguisher isn't solely about projecting water a certain distance; it also plays a crucial role in shaping the spray pattern. In confined spaces, increased pressure can alter this pattern, reinforcing the importance of adjusting extinguisher application based on environmental factors for optimal performance. Understanding the nuances of pressure influence deserves continued study.
7. **Cooling Time and Temperature**: The time needed for effective cooling depends on the initial temperature of the material. Higher temperatures naturally necessitate longer cooling durations, reminding us that a strategic, calculated approach is essential for ensuring complete suppression. More research is needed on ideal cooling times related to different material and fire types.
8. **Environmental Temperature Impacts**: Higher ambient temperatures can affect water's viscosity, potentially altering its flow and overall cooling performance. This underlines the importance of considering environmental factors before employing water-based fire suppression systems, and highlights the need for more research on different environmental impact assessments.
9. **Steam's Oxygen Displacement**: Water's conversion into steam, facilitated by high pressure, isn't simply a cooling mechanism; it leads to a dramatic expansion of volume. This expansion effectively pushes away oxygen, crucial for extinguishing rapidly spreading fabric fires. While the effects are apparent, more data on this aspect could be valuable.
10. **Water's Multifaceted Role**: During a fabric fire, water functions as both a coolant and an oxygen-displacing agent. This multifaceted approach reveals the complexity behind what might appear to be a simple fire suppression technique. However, there are likely more facets to this dual role, which warrants continued study.
The exploration of advanced cooling mechanisms in firefighting highlights the complex interplay of physical and chemical processes. While the principles seem straightforward, understanding these processes at a more granular level opens doors to potential innovations in fire suppression technology. It seems that much can still be discovered through careful and dedicated study.
More Posts from :